Neuroscience Workflow
The right hypothesis and perfect research environment are the keys for solid publications and improvement of translational research. Concurrent imaging and field monitoring provide the perfect research setting for artifact-free MRI images you need. We leave you to choose the perfect hypothesis.
- How many times have you dealt with artifacts that you were not able to correct because you did not know the source of them?
Image artifacts have many causes, are often transient, and are difficult to diagnose. How much do artifacts impact images and how much do they hold back your research? - How many times did you just run your analyses on your standard acquisition just because it was already implemented but not optimized for you?
Standard imaging sequences are designed to meet a wide array of needs and often make compromises, what would it mean if you could have sequences that are optimized for your applications or access to consensus sequences (i.e. HCP)? - How many times did you need to compromise scanning time, patient/participant comfort and image quality?
Imaging studies are subject to many constraints: available scan time, subject comfort and compliance, and scanner capabilities. These constraints can all negatively impact image quality and study results.
We have a solution for you: the NeuroCam™, together with skope-i™ allows you to acquire your neuroimaging data free from corruption.
Improve SNR
The signal to noise ratio (SNR) of an image is one factor in determining the suitability of an image for neuroimaging research use. Improving SNR for neuroimaging means
- fewer scans are lost to poor signal
- more reliable quantitation
- potentially improved statistical analysis*
SNR is a function of both scanner based and physiologically based factors. Scanner based influences that negatively impact SNR often result from transient magnetic field fluctuations during acquisition. These fluctuations can simply reduce the signal or cause the signal to show up in ‘the wrong place’ in the image, which is commonly called ghosting. Field monitoring can help improve SNR by directly measuring magnetic field fluctuations and accounting for them during image reconstruction.
Field monitoring with the NeuroCam and skope-i means that these fields are directly measured and accounted for automatically, without the need for you to spend time implementing your own image reconstruction or resorting to post-reconstruction methods that only estimate the errors. Automated, direct measurement ensures that your images best reflect subject physiology.
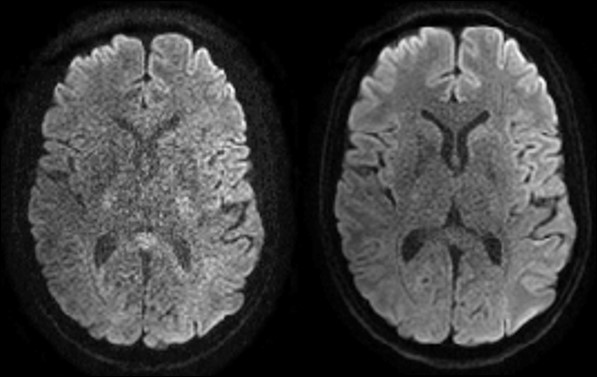
Courtesy of Klaas Prüssmann and group, IBT ETH Zurich and University of Zurich
Twice refocused spin echo sequences have been the mainstay of diffusion neuroimaging because the long delay between diffusion encoding and image acquisition reduces eddy current artifacts. With field monitoring, eddy current effects are measured and accounted for automatically, allowing for higher SNR single refocused spin echo sequences to be utilized in imaging studies.
Reduce artifacts
Artifacts are a result of unwanted scanner or physiologically derived disruptions in the image acquisition process. These disruptions cause data to either be lost (low signal), placed in the wrong location (ghosting and geometric distortion). Reducing artifacts in neuroimaging allows for a more faithful recreation of the physiology or pathology being imaged. Artifacts can disrupt quantitative analyses and pre-processing steps common to neuroscience studies such as image registration and intensity correction.
Many classes of artifacts are caused by transient fluctuations in the magnetic field of the scanner during image acquisition. Correction of these fluctuations can be estimated by codes which assume a model of the scanner’s operation or by direct measurement of the fluctuations. Direct measurement using the NeuroCam requires no assumptions about scanner operation, recording fluctuations from both scanner and physiologically derived sources such as eddy currents and the cardiac cycle, respectively. Reconstruction of images using skope-i produces images corrected of many types of image artifacts.
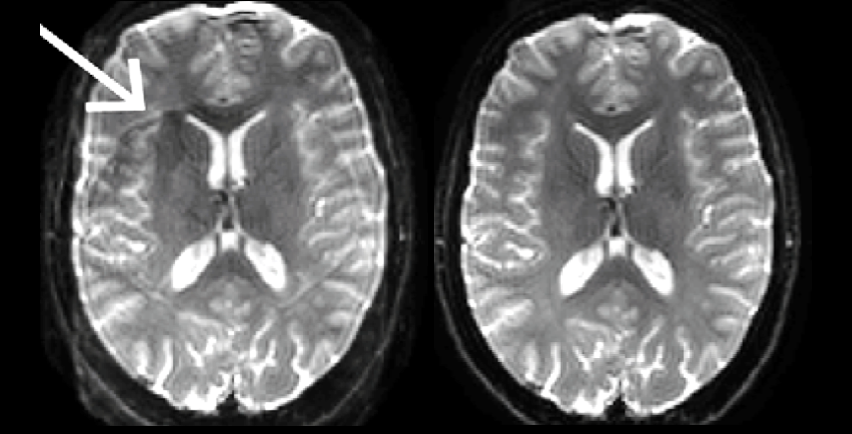
Courtesy of Klaas Prüssmann and group, IBT ETH Zurich and University of Zurich.
Spiral Diffusion imaging
Diffusion imaging is one of the most commonly used sequences in neuroimaging today. It is used to measure tissue microstructure, identify pathologies, and provides the data for tractography. The SNR of diffusion images can be a determining factor in the size of a neuroscience study and can impact the effect size measurable in a study. Improving the SNR means that studies can potentially have greater statistical power and generate more accurate, meaningful results. Alternatively, spiral be used to acquire images with higher resolution, allowing for a more detailed view of neuroanatomy and pathology.
Diffusion SNR is impacted by a wide range of choices made at the scanner console. The choice of the proper ‘pulse sequence’ for your application is one of the most important decisions you will make. Utilizing a ‘spiral’ sequence can improve the SNR by more than 40% when acquired with the NeuroCam. Spiral imaging has historically been difficult because of various artifacts. The NeuroCam, with it’s built in field monitoring capability is able to measure those artifacts and deliver images that harness the full improvement in SNR afforded by spiral diffusion imaging.
Diffusion imaging acquired with spiral readouts holds potential for clinical applications and neuroscience as the improved SNR can translate into higher resolution without averaging. For sub-millimeter resolution spiral readouts allow the reduction of the number of averages and therefore scanning time without compromising quality.
Lee et al. demonstrated the benefits of spiral readouts for diffusion imaging on a standard clinical 3T scanner. Their results demonstrate that spiral acquisitions had higher SNR than all of the EPI methods tested (image shows their spiral implementation versus the traditional Stejskal-Tanner and twice refocused spin echo EPI implementations).

fMRI application
With the NeuroCam, Spiral fMRI is the key solution for applications that require high spatiotemporal resolution such as laminar or columnar fMRI and for standard neuroscience applications. In fact, sub-millimeter resolution was demonstrated with high depiction quality and anatomical congruency.
NeuroCam allowed the acquisition of a single-shot spiral BOLD fMRI at 0.8mm in-plane resolution delivered competitive image quality and BOLD sensitivity (https://doi.org/10.1101/842179).
Once the fMRI spiral is set, analysis pipeline is equivalent to standard fMRI.
Preprocessing steps using SPM12 including slice-timing correction, realignment, co-registration, bias-field correction and smooth with a Gaussian kernel of 0.8mm FWHM, i.e., the voxel size of the high-resolution scan. A general linear model (GLM) contained regressors for the task (visual stimulus) convolved with the hemodynamic response function (HRF), as well as nuisance regressors for motion (6 rigid-body parameters) and physiological noise regressors.
![]() |
With 0.8 mm nominal resolution you can differentiate between significant activation sites during a visual task in neighboring voxels within gray matter only.
![]() |
![]() |
NeuroCam as standard Brain Coil
In case you do not want to add the field monitoring, NeuroCam can simply be used as a head coil with your standard protocol as it also has excellent SNR as a receive coil.
![]() |
Courtesy of IBT ETH Zurich and University of Zurich
Here are results for an fMRI finger tapping task. FMRI data were analyzed using the standard protocol from SPM12 and overlayed on an anatomical T1 MPRAGE acquired with the NeuroCam.
![]() |
